Article of the Month -
August 2007
|
High-Sensitivity GPS - the Low Cost Future of
GNSS ?!
Volker SCHWIEGER, Germany
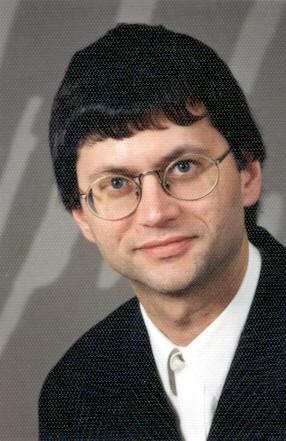
This article in .pdf-format.
SUMMARY
In general it has to be distinguished between GPS receivers and
evaluation techniques used by surveyors, and the mass market solutions. Main
difference is the accuracy that can be reached. During the last years two
developments have taken place that may change the attitude of a surveyor
regarding this separation. On the one hand research and implementation
regarding use of low cost GPS receivers for geodetic purpose is on the way.
In future this may lead to a merger of these two application fields. On the
other hand the mass market gets more interesting as a working field for
surveyors. The latter is connected with the catch words wireless-assisted
GPS and more recently high-sensitivity GPS. These new technologies allow to
establish a higher availability of GNSS signals in urban canyons or even
indoor. An overview about these technologies is given in this paper as well
as an insight into the respective market.
The paper deals with quality characteristics like availability and
accuracy of high sensitivity GPS receivers as well as navigation receivers.
Recent results obtained at University Stuttgart are presented. As expected
availability increases, but accuracy is reduced, if low quality signals are
tracked. Additionally the possibilities for the geodetic community to reach
“geodetic” accuracies are outlined based on new results using low cost
receivers.
ZUSAMMENFASSUNG
Im Allgemeinen ist zwischen hochgenauen GPS Empfängern und
Auswertetechniken und den Massenmarktlösungen zu unterscheiden; insbesondere
aufgrund der erreichbaren Genauigkeit. In den letzten Jahren haben zwei
Entwicklungen stattgefunden, die die Einstellung des Vermessers hinsichtlich
dieser Trennung ändern könnten. Einerseits wird der Einsatz von Low Cost GPS
Empfängern für geodätische Anwendungen erforscht. Andererseits steigert sich
die Attraktivität des Massenmarkts für den Vermesser. In diesem Zusammenhang
spielen die Schlagwörter wireless-assisted und high-sensitivity GPS eine
große Rolle. Diese neuen Technologien erlauben eine höhere Verfügbarkeit von
GPS Signalen in Straßenschluchten oder sogar innerhalb von Gebäuden. Der
Beitrag gibt sowohl einen Überblick über diese Technologien als auch einen
Einblick in die jeweiligen Marktsegmente.
Der Beitrag beschäftigt sich mit Qualitätsmerkmalen wie Verfügbarkeit und
Genauigkeit von High-Sensitivity GPS Empfängern und Navigationsempfängern.
Wie erwartet steigt die Verfügbarkeit bei gleichzeitiger Reduktion der
Genauigkeit, wenn schwache GPS Signale genutzt werden.
1. GNSS MARKETS
Surveyors use the Global Positioning System (GPS) as well as the other
active or planned Global Navigation Satellite Systems (GNSS) as tools for
precise positioning. The active Russian GLONASS and the planned European
Galileo are seen as improvements to gain accuracy for positioning in the 10
cm up to 1 mm level. Additionally the realtime capability is of importance
for the surveyors due to economic reasons. This attitude leads to a focus on
the market for high precise and high price (more than 10 000 €) positioning
receivers that use the carrier phase for positioning. This market is
controlled by some manufactures that provide high quality receivers and
antennas as well as realtime or post-processing software (e.g. Leica
Geosystems, Trimble, Topcon). As a typical example the SmartStation of Leica
Geosystems is presented in figure 1. The European commission predicts the
GNSS market share for geodetic applications to 1 %.

Fig 1: Leica SmartStation (left) and Garmin StreetPilot (right, source:
Garmin)
Most of the GPS (and in the future GNSS market) is not within the focus
of the surveyors: traffic applications, location-based services, navigations
markets, recreation and touristic markets. The receivers and antennas used
for these markets are integrated into car navigation systems or mobile
phones to create services often called location based services. The
technology is hidden behind the service respectively the application. The
user often even does not know that he uses GPS technology, since he is
interested in the result only. This market follows a rushing development
regarding the decrease of investment costs for the users as well as the
increase of quality. Here the predicted market share reaches more than 85 %.
Figure 1 presents a typical product of the navigation industry, the Garmin
StreetPilot. The market for navigation type receivers shows some global
players like Garmin or Magellan, but the markets for integrated systems as
well as for manufactures of receivers boards or chip sets like SiRF or
u-blox are much more dissected. In contradiction to high precise positioning
receivers the price for chip sets is clearly below 100 € and navigation
receivers bay me purchased beginning with 100 € too. Here the quality focus
is much more on availability than the accuracy of the position. The
increased number of satellites due to the additional active or planned GNSS
play an important part for this market segment. One additional important
development has to be noticed: the phase data is part of the internal
processing and, in any case for some of the chip set providers, part of the
output data too. This should enable the use of chip sets for geodetic
purpose in the future.
One important change in the domain of satellite positioning is be the
shift from pure GPS positioning to GNSS positioning. This development is and
will be based on two facts. On the one side the start of new GLONASS
satellites made the Russian satellite system a valuable support to the GPS.
The providers of GPS receivers react on this improvement by the integration
of GLONASS into their hard- and software products. On the other side the
European commission together with the ESA develops the European satellite
navigation system Galileo that should be operable in 2008 (EUC 2001). Due to
these facts a distinct improvement of GNSS availability will be the result
(EISFELLER & SCHÜLER 2006). A detailed discussion of these effects is beyond
the scope of this paper.
The paper will outline recent trends regarding GNSS technologies in
chapter 2. Chapter 3 deals with the possibilities to improve the
availability of GNSS due to these new technologies and chapter 4 presents
results regarding GNSS accuracy improvement using receivers working on the
base of the presented technologies. The effects of these quality
improvements on the working field of the surveyors is outlined at the end of
the paper.
2. NEW GNSS TECHNOLOGIES
2.1 High-Sensitivity GPS
An implementation step towards improvement of availability of GPS resp.
GNSS is the development of High-Sensitivity (HS) GPS receivers. The GPS
Interface Control Document (ARINC, 2000) defines the minimum GPS signal
strength for a user on the earth surface. For c/a-code this is defined with
-160 dBW. The unit dB indicates a logarithmic power ratio with the and as
power values to be compared
.
In the case defined above the unit dBW describes a power ratio with respect
to 1 Watt
.
The defined value on the earth surface leads to the transmitting signal
power for the c/a-code at the satellite to 14.3 dBW that equals 27 Watt (W).
Using the transmitting power given before, the value of -160 dBW may be
reached only, if no attenuation occurs. In reality the GPS signals are
attenuated e.g. by the atmosphere, trees, buildings. According to WIESER &
HARTINGER (2006) the attenuation may reach values about 5dB in cars, up to
20 dB in buildings and more that 25 dB in subterranean garages. These
attenuation factors lead to the problems that occur with the acquisition of
GPS signals inside buildings. “Normal” GPS receivers, especially GPS
receivers for geodetic applications, do not work in-door, because the
sensitivity is not sufficient to track signals with low dBW values.
If low cost one frequency receivers are concerned, most providers sell
receivers that acquire signal below -180 dBW. These receiver type is called
high-sensitivity receiver. In the following it will be called HS GPS. These
high-sensitivity receivers reach an improved signal-to-noise ratio (SNR).
Due to the fact that the signal power may not be changed, the noise power
that is a function of bandwidth and noise density, has to be reduced to
improve the SNR. For this task a longer integration time for the acquisition
of a signal is required to reduce the effect of the noise on the correlation
process for the c/a-code. This is not possible due to the limitation by the
Doppler effect as well as the length of the data bits of the navigation
message. This may be overcome, if non-coherent integration is used
additionally. Nevertheless the possibility to acquire signals with low dBW
values may be realised only in hours. Due to this reason HS receivers use
many correlators to reduce the search space for each correlator. Typical
recent values are e.g. 200 000 correlators. In contradiction “normal” GPS
receivers work with 36 correlators only. A good overview and more details
are given e.g. in WIESER & HARTINGER (2006). Sometimes the integration time
is still to large, especially if the attenuation is to large. The problem
may be solved, if external information are available aiding the receiver to
reduce the search window for each correlator. These external information are
provided using so-called wireless assisted GPS described in the following
section.
2.2 Wireless Assisted GPS
The integration of external information is called wireless assisted or
more simple assisted GPS. As ascertained by WIESER & HARTINGER (2006)
external information are required, if the carrier-to-noise-power
density-ratio ( ) is below 28 dBHz. The is defined as the product of SNR in
dB and integration time in seconds. The possible information to aid the
receiver are the position of the receiver antenna, the positions of the
satellites, the velocities of the receiver as well as the ones of the
satellites and the time information. All these information will aid the
receiver to know where to search for the satellites. The positions of the
satellites are included in the satellite ephemeris. This is valid for the
time information and indirectly the satellite velocities too. In general
these information are included in the navigation message. To load down this
message the receiver needs contact to at least one satellite 12.5 minutes
(for ephemeris 30 seconds is sufficient) without any interruption. This is
often not possible in the case of cold start of a GPS receiver in an
environment with strongly attenuated signal like inside a building. The
problem may be overcome by transmitting the navigation message that is
acquired at another location to the GPS receiver. One possibility to do so
is the transmission via mobile phone communication e.g. GSM or UMTS.
Additionally the position of the receiver antenna may be determined and
transmitted to the GPS receiver. One possibility to estimate the position of
the GPS receiver is the use of mobile phone positioning technology (e.g.
WUNDERLICH et al. 2006). It has to be remarked that velocities of the
receiver antenna may be determined by different methods too. The
transmission via GSM is the reason that one talks about “wireless assisted
GPS” or shorter “assisted GPS” (A-GPS). CARVER (2005) shows the improvement
that can be reached by different aiding information starting from ephemeris
information only, up to time, ephemeris, receiver position and satellite
velocities expressed in Doppler values. He presents gains from 165 dBW for
pure ephemeris aiding up to 187 dBW for the last step using all aiding
information within the same required acquisition time.
WUNDERLICH at al. (2006) distinguishes between network-based A-GPS, where
the measured data is transmitted to a central computing station, from where
the receiver position is transmitted back to the mobile user, and
network-assisted, where the GSM-GPS-combination determines the position off
the GPS receiver on the GPS receiver. In both cases the aiding information
is send via mobile communication to the mobile user before. Up to now the
establishment of such central computing centres is not realized all over the
world; e.g. in Europe the intention to implement services based in the
mentioned techniques is low, since data privacy is difficult to ensure.
First results are obtained e.g. in USA (CARVER 2005, GARIN et al. 1999).
3. AVAILABILITY IMPROVEMENT
3.1 General Information
The availability of GPS may be improved by an improved sensitivity,
meaning the acquisition of signals with a low dBW value, using GPS receivers
(HS GPS) in combination with aiding information (A-GPS) or without this
information. Besides, the availability may be improved by the use of signals
of Galileo and GLONASS. The second possibility is not discussed in this
paper, since experiments are not available.
Recently some investigations using HS GPS are presented that discuss
availability and accuracy of this technology. WIESER & HARTINGER (2006)
report availability improvements up to 60 % in build up areas. The accuracy
is documented with 95%-confindence regions from 1.4 m for points without
shadowing effects up to 142 m for the built up area. LACHAPELLE (2004)
determines a 75 m 95%-confindence region inside a commercial building.
EISSFELLER at al. (2005) estimated three-dimensional standard deviations of
about 25 m for the transition between indoor and outdoor environment. Indoor
they achieved standard deviations of up to 31 m. For the indoor environment
they admit that the standard deviations are repeatability standard
deviations not referred to known coordinate values.
The high availability as well as the accuracy values are confirmed by
investigations that deal with the potentiality of navigation receivers.
These receiver type is frequently equipped with high-sensitivity chips.
Among others RAMM & SCHWIEGER (2004) obtain availability rates up to 100 %
and three-dimensional standard deviations of 7 m even under dense canopy for
the best tested navigation receiver.
3.2 Recent Results at IAGB
To investigate the availability and accuracy of HS GPS receivers
available on the market the IAGB purchase three chip sets including the
respective evaluation kits. Table 1 gives an overview about the
characteristics of the three receivers SiRFstarIII, u-blox LEA-4T and
Fastrax iTrax 03 as given by the manufacturers. The three receivers fulfill
the HS requirement to track signal below -180 dBW. The differences in
tracking sensitivity are small. All of them may be used for A-GPS too. Due
to the lack of A-GPS central servers in Germany it is not possible to
present results regarding this technique. In the last column of the table
the possibilities to get the phase data out of the receiver are presented.
Obviously only the u-blox LEA-4T may be used for this application. In
chapter 4 the author will deal with this topic.
Tab. 1:
Characteristics of tested HS GPS receivers
Receiver |
tracking sensitivity |
A-GPS |
output of phase data |
SiRFstar III |
-189
dBW |
possible |
only
with special agreement |
u-blox LEA-4T |
-188
dBW |
possible |
yes |
Fastrax iTrax 03 |
-186
dBW |
possible |
not
available |
For the investigations a special mount for centering the three receivers
is developed. Figure 2 shows the three antennas mounted on the adapter. The
research regarding availability and accuracy of receivers is carried through
within a study thesis at IAGB; it is still ongoing. The measurements are
carried through for environments with different shadowing effects. Each
measurement is carried through for 20 up to 30 minutes. The three GPS
receivers are working at the same time and the respective navigation
solutions are stored on a computer. The “true coordinates” used for
determination of the standard deviations are measured and estimated by
precise geodetic instruments (PDGPS and tachymeter) and methods. In the
following the author presents the results for the scenarios “free horizon”,
“extreme multipath” and “indoor”. Figure 2 shows the environments for the
latter two scenarios. Figure 3 presents an exemplary plot of the deviations
of the measured coordinates from the true coordinates for the scenario
“indoor”. The red cross on the left site indicates the true position of the
point. On the right site of figure 3 the time period, in which the window
(compare figure 2 right) was closed is clearly visible, because no data is
available in this time. The right as well as the left site of figure 3 show
some outlyers of more than 100 meters in the mentioned time period.

Fig. 2:
Photos of scenarios “extreme multipath” and ” indoor”

Fig. 3: Exemplary deviation plots for scenario “indoor”; horizontal
deviations (left) and horizontal and vertical deviations versus time (right)
of receiver 1
Table 2 presents the availability and the accuracy results for the
different scenarios. The availability is given in percentage of all possible
measurements for the measured time period. For the accuracy reproducibility
standard deviations (RMS) are given. The three scenarios, the three
receivers (Rcv. No.) and horizontal, the vertical and the three-dimensional
(3D) RMS are listed. Additionally the repeatability standard deviations
(precision) are given for the three-dimensional case. The difference between
3D RMS and 3D precision visualizes the influence of systematic effects. The
three receivers are named by one, two and three to avoid discussions with
the manufactures for the time being, since the results are intermediate.
Tab. 2:
Accuracy and availability for different scenarios and receivers
Scenario |
Rcv.
No. |
RMS
[m]
horizont. |
RMS
[m]
height |
RMS
3D [m] |
Precision
3D [m] |
Availability
[%] |
“free
horizon” |
1 |
2.59 |
5.57 |
6.34 |
1.86 |
100 |
2 |
3.90 |
4.71 |
6.32 |
2.51 |
99 |
3 |
4.24 |
2.01 |
4.74 |
2.77 |
43 |
“extreme multipath” |
1 |
26.15 |
25.61 |
36.71 |
10.12 |
100 |
2 |
22.62 |
22.16 |
31.67 |
9.69 |
83 |
3 |
32.18 |
18.29 |
37.42 |
26.96 |
84 |
“indoor” |
1 |
26.02 |
33.97 |
42.79 |
22.29 |
87 |
2 |
36.71 |
36.04 |
51.45 |
38.73 |
48 |
3 |
56.30 |
44.08 |
71.51 |
54.93 |
14 |
Table 2 makes clear that in general the availability and the accuracy
decreases for environments with attenuated (indoor) and reflected signals
(extreme multipath). The receiver 1 delivers the best results, even for
indoor environments. For this receiver a 3D RMS of 43 m could be reached.
The other receivers get a worse RMS, but the results still coincide with the
ones presented in chapter 3.1. Although the sensitivities of the three
receivers were specified almost indentical by the manufactures, the
availability is quite different: from 87 % to 14 % for the “indoor”
scenario. Here further investigations have to be carried through in the
future. Nevertheless “normal” GPS receivers would not get any position
solution for the scenarios “extreme multipath” and “indoor”, so that it may
be concluded, that any of the HS receivers lead to an improvement of
availability.
3.3 Conclusions
The general information as well as the intermediate results at IAGB point
out that the use of HS GPS leads to a gain in availability. Measurement in
urban canyons as well as inside buildings are possible. The accuracy
decreases in the case of reflected and/or attenuated signals e.g. inside
buildings. Nevertheless the acquired positions may be used for many mass
market applications, but they are not usable for geodetic purpose.
4. ACCURACY IMPROVEMENT
4.1 General Information
A clear improvement of accuracy for GPS positioning is possible only by
the use of phase data. The literature documents differences in accuracy
between code and phase solutions with a factor of approximately 100 (e.g.
SCHWIEGER 2003). In principle almost all GPS receivers use the phase data.
Low cost receivers like navigation type receiver, chip sets or integrated
receivers use the phase only to smooth the code observations and in the
following to smooth the estimated position over time. In general the phase
data are not available to the user. The recent HS GPS receivers, like the
ones mentioned in table 1 in chapter 3, have the possibility to extract the
phase data using a documented interface. Unfortunately the interface is not
activated e.g. for SiRF chips like the SiRFstarIII. It is a premium option
not available to the public or to universities. But other manufactures like
u-blox provide this possibility e.g. for the LEA-4T, so that the phase data
can be used for positioning in realtime or post-processing. Currently the A.
Wieser of the TU Graz uses the phase data for investigations regarding
monitoring of landslides. The IAGB plans some research for the future, too.
As written before HS GPS chips are implemented in different applications
like car navigation systems or navigation type receivers. For example SiRF
chips are integrated into several Garmin receivers. This is the reason that
results obtained with navigation receivers are comparable to results
obtained with HS GPS receivers. HILL et al. (2001) presented first results.
Recently ABIDIN & MUCHLAS (2005) reach standard deviations below 20 cm for
baselines up to 100 km length and 20 minutes measurement time.. In the
following the author will focus on investigations using Garmin eTrex Vista
receivers that are equipped with a SiRFstarII chip. At IAGB several
investigation have been carried through (SCHWIEGER 2003, SCHWIEGER & GLÄSER
2005, SCHWIEGER & WANNINGER 2006) to emphasise on the possibilities of
accuracy improvement.
4.2 Recent Results at IAGB
In SCHWIEGER & GLÄSER (2005) some investigations using Garmin eTrex Vista
receivers were presented. It is referred to the same publication for a
detailed explanation regarding the used instruments and equipment as well as
the estimation algorithms. In SCHWIEGER & GLÄSER (2005) the remaining
problem was the solution for half ambiguities and cycle slips. Due to this
reason the software Wa1 developed by Lambert Wanninger was adapted to this
problem. The following chapter presents extracts of SCHWIEGER & WANNINGER
(2006), who solved the problem by the adapted WA1 software.
In the following a brief introduction is given. Figure 4 shows the pillar
network of University Stuttgart in Stuttgart-Vaihingen. The coordinates are
known with standard deviations at mm-level. The measurements with the Garmin
receivers have been carried through in 2004. One Garmin eTrex Vista receiver
has been placed on pillar 6. The second receiver was used on the pillars 1,
4, 5, 7 und 10. Figure 4 shows the measured baselines too. All off them were
measured approximately 30 minutes. The baseline lengths accounts up to 1.2
km. Pillar 1 is shadowed by trees and pillar 10 is located in the vicinity
of a building. For these two pillars shadowing, multipath and diffraction
effects are expected. The characteristics of the baselines are summarized in
table 3.

Fig. 4:
Pillar network in Stuttgart Vaihingen and measured baselines
(SCHWIEGER & WANNINGER 2006)
Tab 3:
Characteristics of measured baselines
pillar |
5 |
4 |
1 |
7 |
10 |
baseline length [km] |
0.12 |
0.26 |
0.45 |
0.45 |
1.1 |
number of satellites |
7 |
8 |
7 |
8 |
6 |
vicinity |
free |
free |
tree |
free |
building |
For baselines without shadowing the solution could be generated
automatically, but for pillars 1 and 10 one respectively two satellites have
to be eliminated during the post-processing. For these steps the residuals
have to be analysed. If the analyse step is not carried through, erroneous
ambiguities lead to errors in the coordinate space of up to 30 cm. This
shows that an automatic error-free estimation of the ambiguities is possible
only in case of further adaption of the Wa1 software to low cost receiver
technology.

Fig. 5: Deviations of measured coordinates from given coordinates
(SCHWIEGER & WANNINGER 2006)
Fig. 5 allows the statement that under the conditions of 30 minutes
measurement time and baseline lengths up to 1.1 km standard deviations of
1.5 cm for the horizontal components and of 1.0 cm for the height may be
achieved.
In SCHWIEGER & GLÄSER (2005) the same baselines were evaluated using the
Leica software SKI-Pro, that could not overcome the problem of half
ambiguities and cycle slips. Figure 6 presents a comparison of the two
positioning results. The three-dimensional deviations to the given
mm-accurate coordinates are given. In general the deviations are clearly
smaller for the Wa1 solutions. This is valid for baselines measured with a
combination of Garmin receivers and Leica receivers too (SCHWIEGER &
WANNINGER 2006).

Fig. 6: Comparison of results of Wa1 and SKI-Pro
(SCHWIEGER & WANNINGER 2006)
The potential of these low cost receivers for kinematic applications is
of market interest too. In the following figure 7 the estimated coordinates
are presented for an exemplary baseline epoch-by-epoch; meaning that the
solution for the coordinates is realised with the respective measurements of
one epoch only. Systematic deviations, that have been estimated, are
eliminated before the estimation of the random deviations is realized. The
precision (repeatability standard deviation) of these solutions shows the
same magnitude like for geodetic receivers for the measured short baselines.
These standard deviations are smaller than 1 cm for the horizontal
components and slightly above 1 cm for the height. These accuracy levels
show that low cost receivers may be used for precise kinematic applications
too, if appropriate software is used.

Fig. 7: Epoch-by-epoch coordinates solutions for baseline between pillars
5 and 6,
medium deviations to given coordinates eliminated (SCHWIEGER & WANNINGER
2006)
4.3 Conclusions
Low cost GPS receivers that are equipped with HS GPS chips may be used
for precise GPS positioning as well as for precise kinematic applications.
The accuracy is at the level of 2 cm for baselines up to 1.1 km and
measurement times of approximately 30 minutes. These results are sufficient
for surveying applications like cadaster and construction works. The results
are obtained in post-processing using an adapted software. For the future
the results have to be delivered more reliable and in realtime to convince
practioners of the low cost approach. Further improvements are expected due
to the fact that the manufacturer of HS chip sets recently provide higher
sensitivity and phase data without the “half-cycle-slip-problem”. The use of
HS GPS for geodetic purpose seems to be possible in the near future.
5. THE FUTURE OF GNSS – MERGING OF MARKETS
The preceeding chapters have shown that on the one side the availability
of GPS signals increases due to new receiver technologies. This will make
the GNSS application possibilities wider within the level of low cost and
low accuracy mass market. The use of additional sensors for positioning gets
less essential. It will be still important for application demanding for
high reliability and availability as security related tasks like aviation
applications.
On the other side the author has shown that the accuracy of navigation
type receivers and therefore mass market HS chipsets may meet the
requirements of a surveyor, if the right evaluation methods are used. This
means that the mass market may influence the surveying community in the next
future. In the extreme case this technology may make special geodetic
receivers obsolete. Than the providing of software would be the only task
for the manufacturers of geodetic GPS respectively GNSS receivers.
The two development lines make the involvement of surveyors into the mass
market essential,
- since the money is made within the low cost sector and not for surveying
applications
- and GNSS surveying applications may be mass market driven in the future.
6. FIG WORKING GROUP 5.4 ACTIVITIES
In the context of this paper the author like to present the activities of
the new established Working Group 5.4 “GNSS” within the Commission 5. Within
this paper an extract regarding the planned Special Study Groups is given.
Due to the importance of the mass market for the surveyors outlined in this
paper, a Special Study Group 5.4.1 “Cost-effective GNSS” is established.
Everybody interested in the topics of this paper may participate in this
group. As a matter of course you are invited to participate in any other
Special Study Group or Working Group within Commission 5 too.
Working Group 5.4 - Special Study Groups
Special Study Group 5.4.1 Cost-effective GNSS
- Analysis of current “low cost” GNSS markets (e.g. LBS, traffic)
including additional necessary technologies like e.g. odometers, mobile
phones
- Recommendations for surveyors to infiltrate these markets
- Development of methods to use “low cost” GPS for precise applications
- Report on cost-effective GNSS
Special Study Group 5.4.2 GNSS networks (together with WG 5.2)
- Analysis and review of GNSS network technologies for practioners
- Analysis of the importance of site calibrations of permanent networks
- Analyse and compare different commercial and non-commercial GNSS networks
- Organise Workshops(s) on GNSS networks
Special Study Group 5.4.3 Precise GNSS
- Develop and publish methods for GNSS heighting
- Develop and publish investigations regarding the GNSS/tacheometer
combinations
- Contact with GNSS equipment manufacturers
- Provide newest technical background information to the practioners
- Develop of input for GPS modernisation and GNSS development
Special Study Group 5.4.4 GNSS calibration and check (together with WG
5.1)
- Development of procedures and recommendations for the check of GPS
equipment
- Recommendations for the calibration of GPS antennas and permanent GPS
sites
- Cooperation with standard organisations (IS0,…)
- Organisation of scientific workshops and tutorials for practioners
- Discussion with GNSS equipment manufacturers about procedures
ACKNOWLEDGEMENT
The author thanks the Ms. Junyu Mao, who performed the measurements and
the computations for chapter 3.2 within the scope of her study thesis.
REFERENCES
ABIDIN, H.Z., MUCHLAS, A. (2005): GPS Surveying using Navigation Type
Receivers. South East Asia Survey Congress 2005, 21-25 November, Bandar Seri
Begawan, Brunei.
ARINC Research Cooperation (2003): Navstar GPS Space Segment, Navigation
User Interfaces. Interface Control Document ICD-GPS-200 Revision
IRN-200C-004, El Segundo, California.
CARVER, C. (2005): Myths and Realities of Anywhere GPS. GPS-World, Vol. 16,
No. 9.
EUC (2001): Galileo, unverzichtbar für Europa, Information of European
Commission (EUC).
EISSFELLER, B., SCHÜLER, T. (2006): Das Europäische
Satellitennavigationssystem Galileo. In: GPS und Galileo. Beiträge zum 66.
DVW-Seminar am 21. und 22. Februar 2006 in Darmstadt, Wißner Verlag,
Augsburg, 2006.
GARIN, L.J., CHANSARKAR, M., MIOCINOVIC, S., NOMAN, C., HILGENBERG, D.:
Wireless Assisted GPS-SIRF Architecture and Field Test Results. ION GPS’99,
14-17 September 1999, Nashville, USA.
HILL, C.J., MOORE, T., DUMVILLE, M. (2001): Carrier Phase Surveying with
Garmin Handheld GPS Receivers. Survey Review, pp 135-141.
LACHAPELLE, G. (2004): GNSS Indoor Location Technologies. The 2004
International Symposium on GNSS/GPS, Sidney, Australia, 6-8 December 2004.
RAMM, K., SCHWIEGER, V. (2004): Low-Cost GPS Empfänger für Anwendungen im
Forstbereich. Flächenmanagement und Bodenordnung, Vol. 66, No. 4.
SCHWIEGER, V. (2003): Using Handheld GPS Receivers for Precise Positioning.
2nd FIG Regional Conference, Marrakech, Morocco, December 2-5.
SCHWIEGER, V, GLÄSER, A. (2005): Possibilities of Low Cost GPS Technology
for Precise Geodetic Applications. Proceedings on FIG Working Week 2005,
Kairo, Ägypten, 16.-21.04. 2005.
SCHWIEGER, V., WANNINGER, L. (2006): Potential von GPS
Navigationsempfängern. In: GPS und Galileo. Beiträge zum 66. DVW-Seminar am
21. und 22. Februar 2006 in Darmstadt, Wißner Verlag, Augsburg, 2006.
WIESER, A., HARTINGER, H. (2006): High-sensitivity GPS: Technologie und
Anwendungen. In: GPS und Galileo. Beiträge zum 66. DVW-Seminar am 21. und
22. Februar 2006 in Darmstadt, Wißner Verlag, Augsburg, 2006.
WUNDERLICH, T., PREIS, S, SU, C. (2006): Wireless Assisted GPS – technische
Möglichkeiten und notwendige Infrastruktur. In: GPS und Galileo. Beiträge
zum 66. DVW-Seminar am 21. und 22. Februar 2006 in Darmstadt, Wißner Verlag,
Augsburg, 2006.
BIOGRAPHICAL NOTES
1983 – 1989 |
Study of Geodesy in Hannover |
1989 |
Dipl.-Ing. in Geodesy (University Hannover) |
1998 |
Dr.-Ing. in Geodesy (University Hannover) |
2003 |
Head of Department “Positioning and Measurement Techniques” at
Institute for Applications of Geodesy to Engineering,
University Stuttgart |
2004 |
Habilitation (University Stuttgart) |
CONTACTS
Dr.-Ing. habil. Volker Schwieger
University Stuttgart
Institute for Application of Geodesy to Engineering
Prof. Dr.-Ing. Wolfgang Möhlenbrink
Geschwister-Scholl-Str. 24 D
D-70174 Stuttgart
GERMANY
Tel.: + 49 711 685 84064
Fax: + 49 711 685-84044
Email:
[email protected]
Web site:
http://www.uni-stuttgart.de/iagb/
